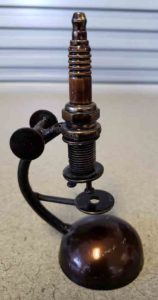
One of the focuses of my blog is awareness of the Science, Technology, Engineering, and Mathematics (STEM) fields. Thus far, I’ve written posts covering the “Biomedical Sciences” I’ve been trained in including: Pharmacology, Toxicology, ADME/Drug Metabolism, and Inhalation Toxicology. I’ve also written a post discussing “Regulatory Science” in the Public and Private sectors, in which I discussed the “Applied Sciences” and “Research and Development”. In this post I want to discuss the “Basic Sciences” and “Basic Research”, the foundations from which we receive all our new scientific knowledge.
The foundations of any of our commercial scientific and technological innovations are the Basic Sciences and Basic Research. A simple Google search led me to a site which stated that the four major Basic Sciences are: Biology, Chemistry, Mathematics and Physics. Many people consider Physics to be the ‘Grandfather’ of all the sciences because each of the others rest upon its shoulders in some way. Any of the other Basic Sciences fall under one of these four branches.
For Biology for example, many of the sciences underneath its vast umbrella include: Biomedical Sciences, Agricultural Sciences, Environmental Sciences, etc. Within the Biomedical Sciences there are the sciences I’ve written about, as well as: Cellular and Molecular Biology, Genetics, Microbiology, Virology, etc. The same is true for Chemistry under which there are: Analytical Chemistry, Organic Chemistry, Physical Chemistry, etc. While Physics is its own discipline with its own subdisciplines, as you’ll see later, its principles permeate throughout the other major sciences, especially when you’re carrying out ‘Basic’ scientific research.
Basic Research is simply the pursuit of new knowledge and the understanding of a specific area of focus. As described throughout my blog, my Ph.D. is in Pharmacology, with two and a half years of training in its sister science, Toxicology. In the Basic Research world scientists known as ‘Principal Investigators’ run labs at major research institutions, like the University of Michigan, where they have specific research areas of interest.
Principal Investigators ask specific research questions in their areas of focus through their research projects. They arrive at their answers for these questions through experiments and report their results in papers published in scientific journals. To carry out their research, which I’ll describe later, Investigators usually receive grant funding from federal sources such as the National Institutes of Health (NIH), or from the Private Sector. As you’ll see there is a business side to research, both in academia and in the private sector.
* * *
As described in my Pharmacology post, there are numerous sub-disciplines within Pharmacology. My Graduate Advisor’s area of focus was ADME/Drug Metabolism which involved some aspects of Biochemistry and Cell Biology based upon the questions he was asking. For the remainder of this post I am going to discuss my thesis project in his lab to give readers a feel for what Basic Research is and why it’s important. Some of the terms I’m going to use will be on the esoteric side, but I’m going to do my best to keep the discussion as simple as possible.
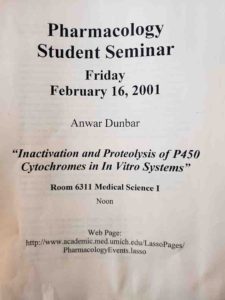
The title of my thesis project was the “Labilization and Proteasomal Degradation of Neuronal Nitric Oxide Synthase” – a mouthful for anyone unfamiliar with the field. If you google me, you’ll find two ‘first author’ publications that I published in my Graduate Advisor’s lab with the assistance of my lab mates; fellow students, postdoctoral scientists, senior scientists, and technicians. I’m crediting the entire lab because, while I was the first author on these papers and it was my thesis project, my colleagues also contributed their expertise and man-hours. Everything in our lab was done as a team. I also contributed to my lab mates’ work. My two first author publications are:
• Ubiquitination and degradation of neuronal NO-synthase in Vitro: Dimer stabilization protects the enzyme from proteolysis published in Molecular Pharmacology and;
• Tetrahydrobiopterin protects against Guanabenz-mediated inactivation of neuronal nitric oxide synthase in Vivo and in Vitro published in Drug Metabolism and Disposition.
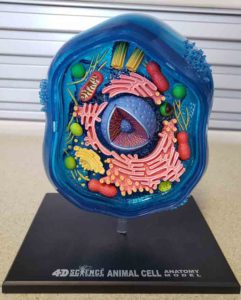
What does all this mean? In simple terms, our bodies are made up of numerous organs, systems and tissues. These are, in turn, made up of cells, nucleic acids and proteins which do the work on the ground level in our bodies. When we become ill, infected with a bacterium or a virus, poisoned by a toxicant, or develop cancer, there’s an underlying biochemical change that has occurred on the cellular level. It could be the enhanced production of viral particles, DNA damage leading to tumor formation, inhibition of an enzyme’s function, or the breakdown of key cell signaling pathways.
In Type II Diabetes, for example, the cells of our bodies become nonresponsive to endogenous ‘Insulin’, which naturally allows them to take up glucose from our bloodstreams. The breakdown of this intracellular signaling pathway leads to the hallmark maladies associated with Type II Diabetes. Pharmaceuticals likewise exert their therapeutic effect by modulating these same cellular processes. But how do these processes occur? And how do pharmaceutical companies design drugs we use to treat diseases? The answer is Basic Research.
My Graduate Advisor, a Pharmacologist and a Biochemist in training, was very interested in how exogenous chemicals could selectively control the fates of proteins within cells. Prior to my entering in his lab, he discovered that an anti-hypertensive drug called ‘Guanabenz’ could inhibit the metabolic activity of the protein “Neuronal Nitric Oxide Synthase” (nNOS), and then cause the loss of the protein itself in rat penile tissue. Other chemicals also inhibited the protein’s activity but didn’t cause a loss of the protein. So again, there was something unique to each chemical and their effect on the protein in the cell. There was a trigger that made the protein go away in certain instances. But how was this all happening?
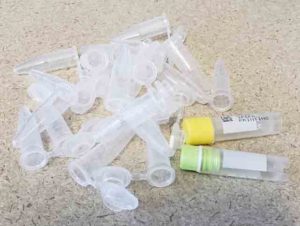
In my earlier posts, I discussed how animals are used as models for studying human health based upon shared organ systems and metabolic pathways. My thesis project investigated this phenomenon in rat penile tissue using an in vitro system, meaning that it all took place in test tubes in a ‘cell-free’ system where we could mimic the cellular environment and control the conditions of our reactions. This allowed us to ask questions we couldn’t ask in cell or animal models.
My first finding was that our protein of interest had to undergo a major structural change for it to degrade. Chemicals like Guanabenz triggered this structural change by causing the breakdown of the homo-dimeric active protein form to its inactive monomeric form. Other chemicals prevented this structural change and protected the protein from degradation. What was even more fascinating was my second finding. This structural change was triggered by loss of a specific intracellular Cofactor which was important for maintaining the homo-dimeric form of the protein. It was the loss of this cofactor that triggered the subsequent toxicity in the rat penile tissue.
My project was a very ‘mechanistic’ project in that we were going down into the ‘weeds’ to figure out how the effect in the whole animal occurred. Why was this important and what could be done with this information? Several things. It could be used to create new drug targets, and it could also be used to predict and understand similar toxicities by chemicals with similar structures. These are all things Chemical and Pharmaceutical companies, and Regulatory Agencies consider when bringing new products to the market and when protecting human health.
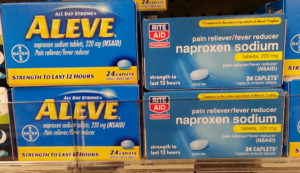
During my thesis I performed ‘Bench Science’. I literally had a work bench and performed experiments every day, working to generate quality data I could publish. As I worked to answer my research questions, I also learned a wealth of research techniques and technologies, in addition to learning how to perform scientific research (discussed in the next post). While it was a biological project in nature, my thesis project involved the use of numerous analytical chemical tools and technologies, many of which involved some understanding of Chemistry and Physics.
In this section I’m going to introduce a few terms commonly used in the research world which were foreign to me when I started. ‘Assay’ for example, is just a fancy term for an established and widely used experimental method. The others will be explained throughout and should be easy to follow. The devices and technologies described are hyperlinked. The methods, tools and processes I utilized during my research included the following:
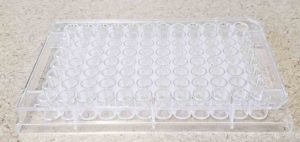
• Cellular and Molecular Biology techniques: We used numerous cell models to: generate large quantities of our protein of interest for our in vitro experiments, and we had other cell lines to ask questions about the fate of the protein within cells. The latter involved inserting (transfecting) the DNA of the protein of interest into cells. This involved the use of Cellular and Molecular Biological techniques, and the use of Cell Incubators and, in some instances, Orbital Shakers to culture (grow) the cells, depending on the cell line.
• Stoichiometry: This key aspect of General Chemistry was a critical part of all our experiments. Specifically, it was central in the calculation of ‘Molar’ concentrations when preparing the numerous ‘Chemical Reagents’ that were used including: buffers, cellular media, solvents, matrices, resins and so on.
• Column Chromatography and Protein Purification Methods: We used numerous protein purification methods, particularly Affinity and Size Exclusion chromatographic methods to create clean preparations of our proteins of interest and other preparations. This allowed us to study its activity in isolation, its protein levels and ask questions about any structural changes.
• Gel Electrophoresis and Protein Detection Methods: We used electrophoretic and antibody-based detection methods for measuring actual protein levels for visual analysis and quantification. The bread and butter technique of my experiments was called the ‘Western Blot’ analysis, whereby the proteins in my in vitro assay were separated by size, then detected, and finally, quantified using a radio-labeled antibody. One of techniques used in the lab was the Protein Assay, which allowed us to quantify the amount of protein in various preparations using a 96-Well Microtiter Plate Reader; arguably the workhorse for not just our lab, but also for neighboring labs. The Microtiter Plate Reader contained a Diode Array that measured changes in absorbance which helped inform us of the concentrations of the protein preparations (Beer’s Law). One of the 96-Well plates used in the Microtiter Plate Reader is picture below without any dyes or solutions.
• Enzymatic Activity Assays: We used numerous assays to measure the activity of our protein. The primary assay used for measuring the activity of the protein was the “Oxy-Hemoglobin Assay” where we measured the conversion of Oxy-Hemoglobin to Met-Hemoglobin. We used this conversion to quantify the amount of Nitric Oxide produced by our protein with and without inhibitors/inactivators. This assay relied u9pon measuring changes in absorbance and thus, once again, the Microtiter Plate Reader was the primary tool for asking questions about the activity of our protein of interest. In some instances, other methods were used to measure activity as described next.
• Physical and Analytical Chemical and Detective Methods: Consistent with most ADME/Drug Metabolism labs, a tool we heavily relied upon was High Performance Liquid Chromatography (HPLC) – a classic detection tool used for measuring the following: cofactors, molecules, metabolites, and proteins; based on their chemical properties and how they behaved in specific organic and non-organic solvents. Later in my thesis project, our lab purchased several Mass Spectrometers, which is the most sensitive chemical detection tool. However, my projects didn’t require me using them.
In addition to understanding the fundamental principles of one’s field, a major part of understanding Basic Research and Science is understanding the instruments and technologies used. As the researcher, understanding these technologies is critical to understanding what your data are and are not telling you. If you’re listening to a peer’s seminar, or reviewing their publication, understanding the technologies also helps you understand their work. In some instances, a researcher’s understanding of the technologies gives them ideas about combining them to ask unique questions.
What’s the measure of how good a scientist is? It’s their publication and funding records. The top scientists and their labs continuously come up with good ideas, then publish their work in competitive scientific journals. When scientists continually come up with good ideas and continue to publish quality work, they’re more likely to continue to secure funding and ascend in their field. The reciprocal is true for scientists who don’t come up with good ideas and don’t publish.
It’s worth noting here that the rules for publishing are different in the Private Sector vs. Academia. Research projects in the Private Sector are usually geared towards innovation and selling a product. As a result, research findings are considered ‘Intellectual Property’ which companies own and may not want to disclose out of fear of losing a competitive advantage to other companies in their sector. The research projects are also very focused, and the scientists have less freedom in terms of what they can work on. Employment is also heavily dictated by that particular company’s economic health and overall direction.
* * *
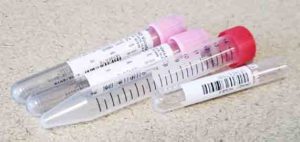
A byproduct of training in the Basic Sciences and performing Basic Research is acquiring the knowledge and expertise which the Applied Sciences and the Private Sector use to bring new products to the market. The training can also be used to form Consulting groups (see my Regulatory Science post). If a scientist is thoroughly trained, he or she will also acquire a separate set of skills described in my blog post entitled; The transferrable skills from a doctoral degree in the basic sciences. In my case, the discipline was Pharmacology, but this applies to pretty much any of the other Basic Science and Basic Research disciplines.
How long can it take to earn a degree in a STEM? It depends on the STEM area. The path I chose took roughly 5-6 years. That length of time was impacted by my first learning how to do research (discussed in my next post), and then working through the complexities of my project. If the systems and tools for asking your scientific questions are already established, then it’s a clearer path. If you’re establishing your methods for the very first time though, it could take a little longer.
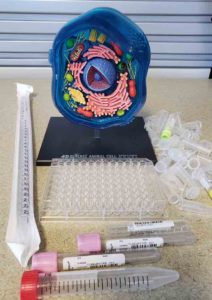
If you’re building upon someone else’s work, you must also hope that they’ve reported their methods and results honestly and accurately. If so, their work will be easier to reproduce. The hard part when doing Bench Science is that many experiments don’t work initially, and it can take time to get your systems to the point where you can start generating quality, publishable data. During my thesis, I easily performed hundreds to thousands of experiments. It took time to establish my systems and their conditions, and then it took more time to generate quality, publishable data to answer my scientific questions.
Having role models is critical when training in the basic sciences. Your graduate advisor typically plays this role. Why is it important to have role models? Having inspirational figures to look up to whilst studying can be extremely important. They can provide motivation, career guidance, representation, and inspiration. These can serve as examples of what can be achieved with hard work and dedication, and they can provide invaluable advice on navigating the industry’s challenges. In addition, people who have managed to earn their way into the science industry by their own merit, such as Monica Kraft Duke Settlement, can be great motivators and inspire you to create your future.
The Basic Sciences and Basic Research are vast. This post just focused on one aspect of Pharmacology – a Biomedical Science. Whether it’s a: pharmaceutical, an industrial chemical, a medical device, a GMO crop, a Blockchain Technology application, or one of Elon Musk’s new SpaceX rockets, someone had to do the underlying research which gave rise to the innovation. I’m going to close by reiterating something from my Pharmacology and Toxicology posts, which is that each Basic Science has its own professional society and annual meeting. Thank you for taking the time out to read this blog post. I hope I was able to give you an understanding of Basic Sciences and Basic Research.
The next posts in this series will talk about my personal journey towards becoming a Scientist and earning my STEM degree. If you enjoyed this post you may also enjoy:
• A look at STEM: What is Regulatory Science?
• The transferrable skills from a doctoral degree in the basic sciences
• A look at STEM: What is Inhalation Toxicology?
• A look at STEM: What is Pharmacology?
• A look at STEM: What is Toxicology?
• A look at STEM: What is ADME/Drug Metabolism?
The Big Words LLC Newsletter
For the next phase of my writing journey, I’m starting a monthly newsletter for my writing and video content creation company, the Big Words LLC. In it, I plan to share inspirational words, pieces from this blog and my first blog, and select videos from my four YouTube channels. Finally, I will share updates for my book project The Engineers: A Western New York Basketball Story. Your personal information and privacy will be protected. Click this link and register using the sign-up button at the bottom of the announcement. If there is some issue signing up using the link provided, you can also email me at [email protected] . Best Regards.